Induced pluripotent stem cells (iPSCs) are emerging as promising tools for modeling and treating human neural diseases.
IPSCs have two main and unique properties: they can self renew indefinitely and they can become any type of cell of an organism - this is why they are called pluripotent. They have properties similar to those of embryonic stem cells (ESCs) but they are produced from adult cells (such as skin cells, for example) rather than from embryos. Indeed, when skin fibroblasts are cultured in a petri dish under the influence of adequate reprogramming factors, they can be reprogrammed into cells that look like ESCs, but which are pluripotent. This seminal discovery by Shinya Yamanaka of Kyoto University was awarded the Nobel Prize in 2012.
“It’s a bit like taking adult cells and making them travel back in time so that they behave like embryonic cells again,” explains Pierre Vanderhaeghen, professor at the Université Libre de Bruxelles in Belgium, AXA Chair in Neurosciences and Longevity. “We can maintain these cells in culture, (suspend them in time if you will) and then allow them to differentiate into other types of cells, such as liver, brain or muscle cells, by placing them in a dish containing specific morphogens (peptides secreted by surrounding cells).”
Credit: L'agence Ody.C for AXA Research Fund
1st surprise: iPSCs can “automatically” transform into cortical cells
The major challenge here is to make iPSCs become a certain type of cell rather than another by using the right culture conditions, but Vanderhaeghen and colleagues discovered that iPSCs and ESCs can differentiate into cortical cells, without being exposed to any particular type of morphogen (Ref: Nicolas Gaspard et al., An intrinsic mechanism of corticogenesis from embryonic stem cells / Ira Espuny-Camacho et al., Pyramidal Neurons Derived from Human Pluripotent Stem Cells Integrate Efficiently into Mouse Brain Circuits In Vivo)
“The cerebral cortex is the most complex structure in our brain and the nerve cells (or neurons) that make it up are crucial elements for its function,” explains Vanderhaeghen. “Indeed, loss of cortical neurons can lead to many neurological diseases, including stroke and Alzheimer’s. You would think that making structures as complicated as cortical cells from iPSCs would require very sophisticated cultures, but, to our surprise, we found that doing this was in fact very simple and that the IPSCs can transform into cortical cells through a ‘default’ pathway. This is because they need to receive little or no molecular signals from morphogens - and this is indeed the way that cells in the embryo become neural cells in nature.”
More surprises: forebrain and cortical cells appear and generate neurons of all six layers of the cortex
The neural tissue formed in this way is, in fact, forebrain tissue (rather than mid- or hind-brain) and this is the tissue from which the cortex emerges. But there were more surprises in store for Vanderhaeghen’s team who also observed that the cells in this tissue could then automatically start to divide into neurons that make up all six layers of the cortex.
“We do not yet know how they do this,” says Vanderhaeghen, “but we do know that they do it in a sequential way, and thus in the same way as the process naturally occurs in the developing embryo. It is important to say that although we can generate neurons corresponding to all six cortical layers in a dish, they do not actually form regular layers (as in vivo) but are rather arranged in a disorganized fashion.”
Nevertheless, we can now study these cortical cells in real time and look at some aspects of cortex development in the lab, he adds. We can also study cortical cells that have been produced from the skin cells of patients suffering from diseases of a genetic origin, such as microcephaly or from neurodegenerative or psychiatric diseases. “All these diseases are little understood because we sometimes lack the right models to study them, which makes it difficult to find a cure.”
Better understanding how the human cortex evolved and developed
The cortical cells produced could also help researchers better understand how the human cortex evolved and developed and why it is so different from that of primates and other animals. (The cortex is said to be divergent – that is, it changed a lot between species during evolution). For these studies, the researchers transplanted mouse-derived and human-derived induced pluripotent cortical cells in a pup mouse brain. They found that the cells can indeed integrate into the animal’s cortex, in very much the same way as they would naturally.
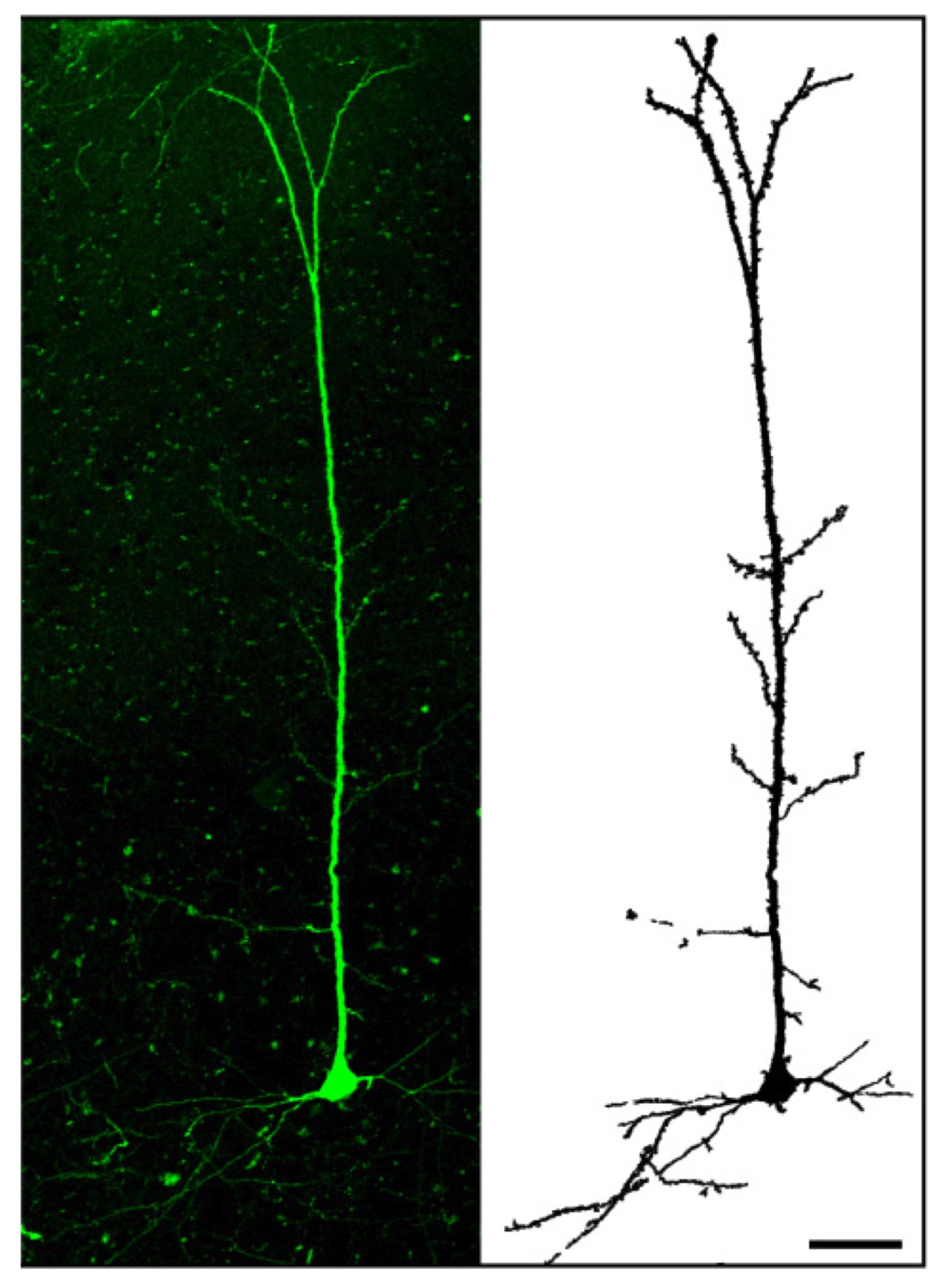
Human cortical neurons transplanted in a mouse brain.Credit: Courtesy of P. Vanderhaegen et al.
“When we transplanted human-derived neurons into the mouse brain, however, we found that they also integrated well, but that they developed at a much slower pace. The mouse neurons took around three weeks to develop whereas the human ones took around nine months. This is strikingly similar to the situation we see in nature: human brains, which are said to be neotenic, develop over decades, unlike mice or ape brains that develop quickly and in the baby animal. This result suggests that the rate of development of neurons is intrinsic to each particular species.”
This slow pace of development could explain why we as humans are good learners and continue developing our intelligence throughout childhood and into adulthood. This is not the case for apes, who although certainly outperform human babies when they are born, quickly flail behind since their development stops early on. Vanderhaeghen believes that some diseases like autism might be caused by impairments in this natural “clock”, but cautions that “this remains speculative at this point”.
Towards brain repair?
The most recent aspect of the group’s work is concerned with potential brain repair – for instance following a stroke or trauma. Here, the researchers transplanted induced pluripotent cortical cells into an adult mouse brain presenting lesions (damage) in the visual cortex and found that the new cortical cells connect to (some extent) the damaged host brain. (Michelsen KA et al., Area-Specific Reestablishment of Damaged Circuits in the Adult Cerebral Cortex by Cortical Neurons Derived from Mouse Embryonic Stem Cells).
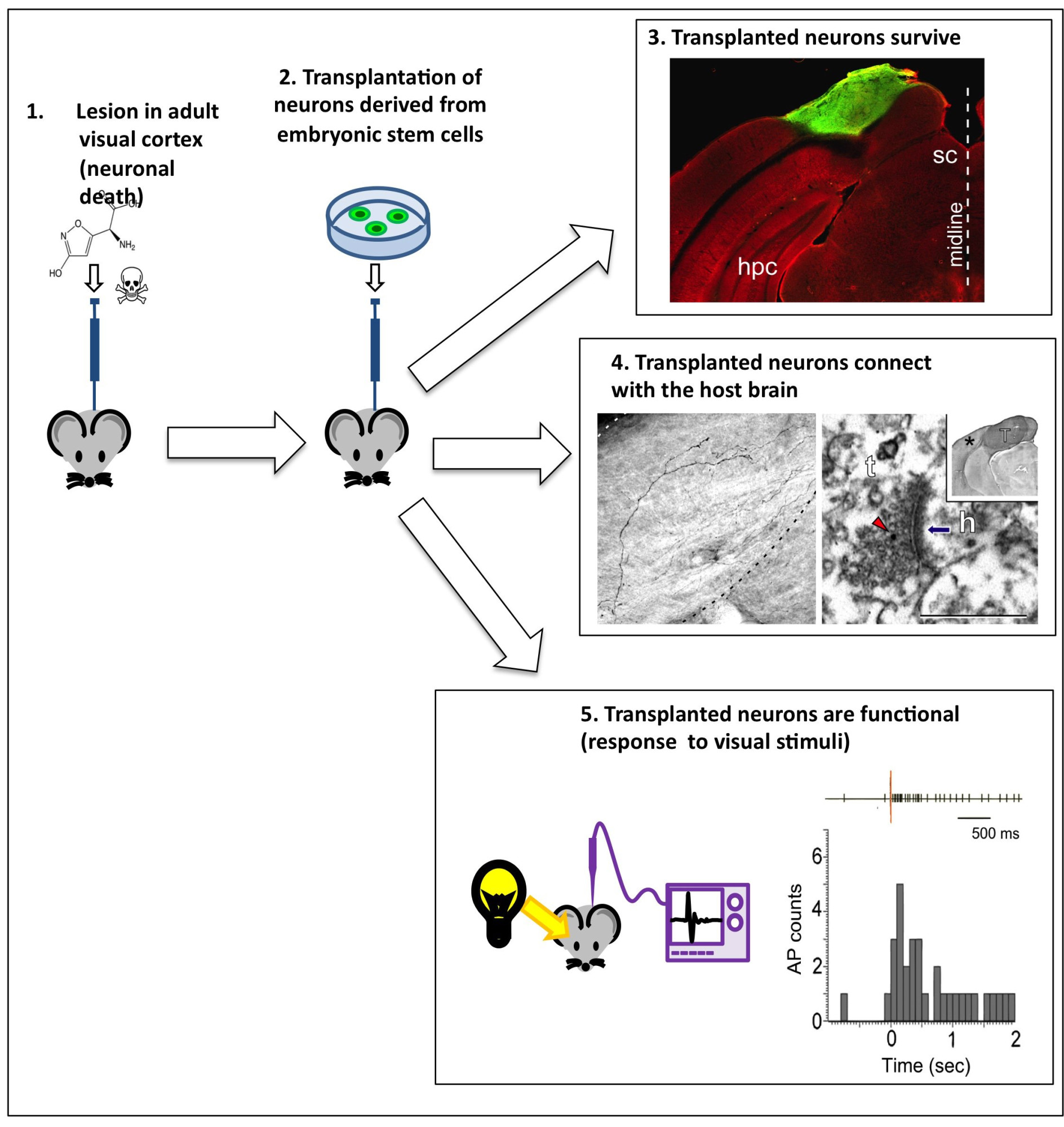
Transplanting neurons into a host brain.Credit: Michelsen KA et al.
“Promising though this result is, there is a lot of work still to be done before we can say for sure whether the new cortical cells could actually repair the damaged regions,” stresses Vanderhaeghen. “We observe that the new neurons are present and connected, and that some of the host cells even respond to visual stimuli (as the visual cortex does) but, since our cortex has not evolved to integrate new neurons, we do not yet know to what extent the new cells will be able to interact with the old ones. Although there is evidence that this is possible in some species (amphibians and fish, for example), there is yet no evidence for this happening in mammals.
“This work is still at the experimental animal model stage and whether the strategy can indeed work in real clinical settings will require a lot more research,” says Vanderhaeghen.
Nonetheless, the experiments have successfully proved that nerve cells can be engineered under controlled conditions and that these cells can be transplanted into damaged cortical circuits. These breakthroughs could ultimately lead to new therapies for patients that have had an ischemic stroke or who are suffering from age-related brain illnesses.
Source : Scientific American
Other articles from the same author
Discover research projects related to the topic
Women's Health
AXA Chair
Spain
Understanding Menopause's Effect on Women's Cardiovascular Risk
Cardiovascular disease (CVD) is the leading cause of mortality for women worldwide, yet women remain understudied. Atherosclerosis, the main pathology... Read more
.thumbnail.jpg)
Inés
PINEDA-TORRA