Climate & Environment
Climate Change
Space & Planetery
Extreme Weather Events
Post-Doctoral Fellowships
United Kingdom
Novel approaches towards understanding stratosphere-troposphere interaction and its role in weather and climate
The dominant modes of variability in the large-scale atmospheric circulation are known as the Northern and Southern annular mode (NAM and SAM) in each hemisphere, respectively. These climate `modes' explain ~30% of the variance in surface temperatures in each hemisphere, and are strongly linked to high-impact weather events such as summer heatwaves (e.g. European summer 2003) and cold and snowy winters (e.g. European winters 2009/10/11). Annular mode (AM) variability is therefore crucial for regional climate. However, the predictability of the AMs on seasonal to interannual timescales is currently poor. Identifying and understanding drivers of AM variability is therefore crucial for improving weather forecasts and climate projections on timescales of weeks to centuries. This project will consider one such driver, namely the stratosphere.
There is now overwhelming evidence that the stratosphere and troposphere behave as a two-way dynamically-coupled system, such that changes in temperature and wind in the stratosphere can influence tropospheric weather patterns, and vice versa. This so-called `stratosphere-troposphere coupling' is an important feature of the Earth system because it presents a pathway for driving variations in surface climate through a number of processes, including the El-Niño Southern-Oscillation, the 11-year solar cycle, stratospheric ozone depletion and increasing greenhouse gas (GHG) concentrations. It is commonly found in climate models and observations that changes in the stratospheric state alter the phase of the tropospheric NAM or SAM. A clear example of this is the Southern hemisphere, where stratospheric ozone depletion has been the dominant driver of the positive SAM trend over the past three decades. This has caused changes in surface temperatures over Antarctica, carbon uptake by the Southern ocean and subtropical precipitation.
The stratosphere is expected to play an important role in the response to anthropogenic climate change in the Northern hemisphere. Climate models which include a good representation of the stratosphere simulate an opposite shift in the North Atlantic storm track compared to 'standard' models with a poorly resolved stratosphere. This has important ramifications for future European weather and the risk of extreme weather events, including heatwaves, wind storms, floods and droughts.
One of the key outstanding challenges is to understand at a process-based level how the stratosphere and troposphere interact. Despite progress over the past decade, the contribution of different mechanisms is still poorly understood. This project will address this issue by using a hierarchy of models and diagnostics to improve our understanding of stratosphere-troposphere interactions. A key issue in climate science is the ever increasing gap between model complexity and the fundamental understanding of a complex and highly non-linear system. This project will bridge this gap by developing a new modeling tool which includes the key components of the climate system needed to investigate stratosphere-troposphere interactions, but which retains sufficient simplicity to diagnose processes and mechanisms.
The model will be based on the Reading Intermediate General Circulation Model (IGCM) and will include simplified representations of the key ingredients for the midlatitude storm tracks, including the continental land masses, topography and sea surface temperature gradients. The first phase of the project (months 1-8) will involve developing and testing the new modeling tool. To achieve this, I will interact with colleagues at Cambridge, the University of East Anglia and the University of Reading who have expertise in using the IGCM. A suite of diagnostics will be developed to identify key processes, including Rossby wave breaking and blocking events, the tracks of synoptic storms and large-scale planetary wave structures.
The new model will provide a framework to conduct experiments during the second phase of the project (months 9-15) to pin-point key processes and mechanisms for stratosphere-troposphere interactions. Particular attention will be paid to the impact of stratospheric perturbations with different zonal structures and positions relative to the storm tracks. This is motivated by an increasing awareness that the structure of a stratospheric perturbation may be important for determining the dynamical impact on the troposphere (e.g. due to ozone depletion, stratospheric sudden warmings). However, many existing studies have used simplified models which do not capture the large-scale zonal structure of the atmosphere. These new experiments will enable key 'sensitive' regions in each hemisphere to be identified for the first time. A key focus will be on the North Atlantic region, where the tilted jet structure brings about a more complex dynamical regime than in other storm track regions.
In the third phase of the project (months 16-21), the idealised experiments will be compared to simulations from the state-of-the-art UKCA coupled chemistry-climate model, which will enable the mechanisms identified in phase II to be tested in the context of stratospheric ozone depletion and anthropogenic climate change.
All phases of the project will benefit from expertise in climate modeling and atmospheric dynamics in the Departments of Chemistry and Applied Mathematics and Theoretical Physics at Cambridge, as well as expertise at the British Antarctic Survey and Scott Polar Research Institute through the newly established Cambridge Centre for Climate Science.
The research will provide new insight into the underpinning mechanisms for how the stratosphere affects weather and climate on timescales of weeks to centuries. There are few components of the climate system which exert a tangible influence over this range of timescales, and it is therefore crucial that we have a detailed understanding of these interactions and the mechanisms by which they take place.
There is now overwhelming evidence that the stratosphere and troposphere behave as a two-way dynamically-coupled system, such that changes in temperature and wind in the stratosphere can influence tropospheric weather patterns, and vice versa. This so-called `stratosphere-troposphere coupling' is an important feature of the Earth system because it presents a pathway for driving variations in surface climate through a number of processes, including the El-Niño Southern-Oscillation, the 11-year solar cycle, stratospheric ozone depletion and increasing greenhouse gas (GHG) concentrations. It is commonly found in climate models and observations that changes in the stratospheric state alter the phase of the tropospheric NAM or SAM. A clear example of this is the Southern hemisphere, where stratospheric ozone depletion has been the dominant driver of the positive SAM trend over the past three decades. This has caused changes in surface temperatures over Antarctica, carbon uptake by the Southern ocean and subtropical precipitation.
The stratosphere is expected to play an important role in the response to anthropogenic climate change in the Northern hemisphere. Climate models which include a good representation of the stratosphere simulate an opposite shift in the North Atlantic storm track compared to 'standard' models with a poorly resolved stratosphere. This has important ramifications for future European weather and the risk of extreme weather events, including heatwaves, wind storms, floods and droughts.
One of the key outstanding challenges is to understand at a process-based level how the stratosphere and troposphere interact. Despite progress over the past decade, the contribution of different mechanisms is still poorly understood. This project will address this issue by using a hierarchy of models and diagnostics to improve our understanding of stratosphere-troposphere interactions. A key issue in climate science is the ever increasing gap between model complexity and the fundamental understanding of a complex and highly non-linear system. This project will bridge this gap by developing a new modeling tool which includes the key components of the climate system needed to investigate stratosphere-troposphere interactions, but which retains sufficient simplicity to diagnose processes and mechanisms.
The model will be based on the Reading Intermediate General Circulation Model (IGCM) and will include simplified representations of the key ingredients for the midlatitude storm tracks, including the continental land masses, topography and sea surface temperature gradients. The first phase of the project (months 1-8) will involve developing and testing the new modeling tool. To achieve this, I will interact with colleagues at Cambridge, the University of East Anglia and the University of Reading who have expertise in using the IGCM. A suite of diagnostics will be developed to identify key processes, including Rossby wave breaking and blocking events, the tracks of synoptic storms and large-scale planetary wave structures.
The new model will provide a framework to conduct experiments during the second phase of the project (months 9-15) to pin-point key processes and mechanisms for stratosphere-troposphere interactions. Particular attention will be paid to the impact of stratospheric perturbations with different zonal structures and positions relative to the storm tracks. This is motivated by an increasing awareness that the structure of a stratospheric perturbation may be important for determining the dynamical impact on the troposphere (e.g. due to ozone depletion, stratospheric sudden warmings). However, many existing studies have used simplified models which do not capture the large-scale zonal structure of the atmosphere. These new experiments will enable key 'sensitive' regions in each hemisphere to be identified for the first time. A key focus will be on the North Atlantic region, where the tilted jet structure brings about a more complex dynamical regime than in other storm track regions.
In the third phase of the project (months 16-21), the idealised experiments will be compared to simulations from the state-of-the-art UKCA coupled chemistry-climate model, which will enable the mechanisms identified in phase II to be tested in the context of stratospheric ozone depletion and anthropogenic climate change.
All phases of the project will benefit from expertise in climate modeling and atmospheric dynamics in the Departments of Chemistry and Applied Mathematics and Theoretical Physics at Cambridge, as well as expertise at the British Antarctic Survey and Scott Polar Research Institute through the newly established Cambridge Centre for Climate Science.
The research will provide new insight into the underpinning mechanisms for how the stratosphere affects weather and climate on timescales of weeks to centuries. There are few components of the climate system which exert a tangible influence over this range of timescales, and it is therefore crucial that we have a detailed understanding of these interactions and the mechanisms by which they take place.
To add or modify information on this page, please contact us at the following address: community.research@axa.com
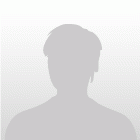
Amanda
MAYCOCK
Institution
University of Cambridge
Country
United Kingdom
Nationality
British
Related articles
Climate & Environment
Climate Change
Post-Doctoral Fellowship
Hungary
How Will Climate Change Affect Bird-Spread Diseases
Expected start date:Aug-2023 Human, animal, and environmental health are interconnected. Climate change may alter the transmissions of diseases that can... Read more
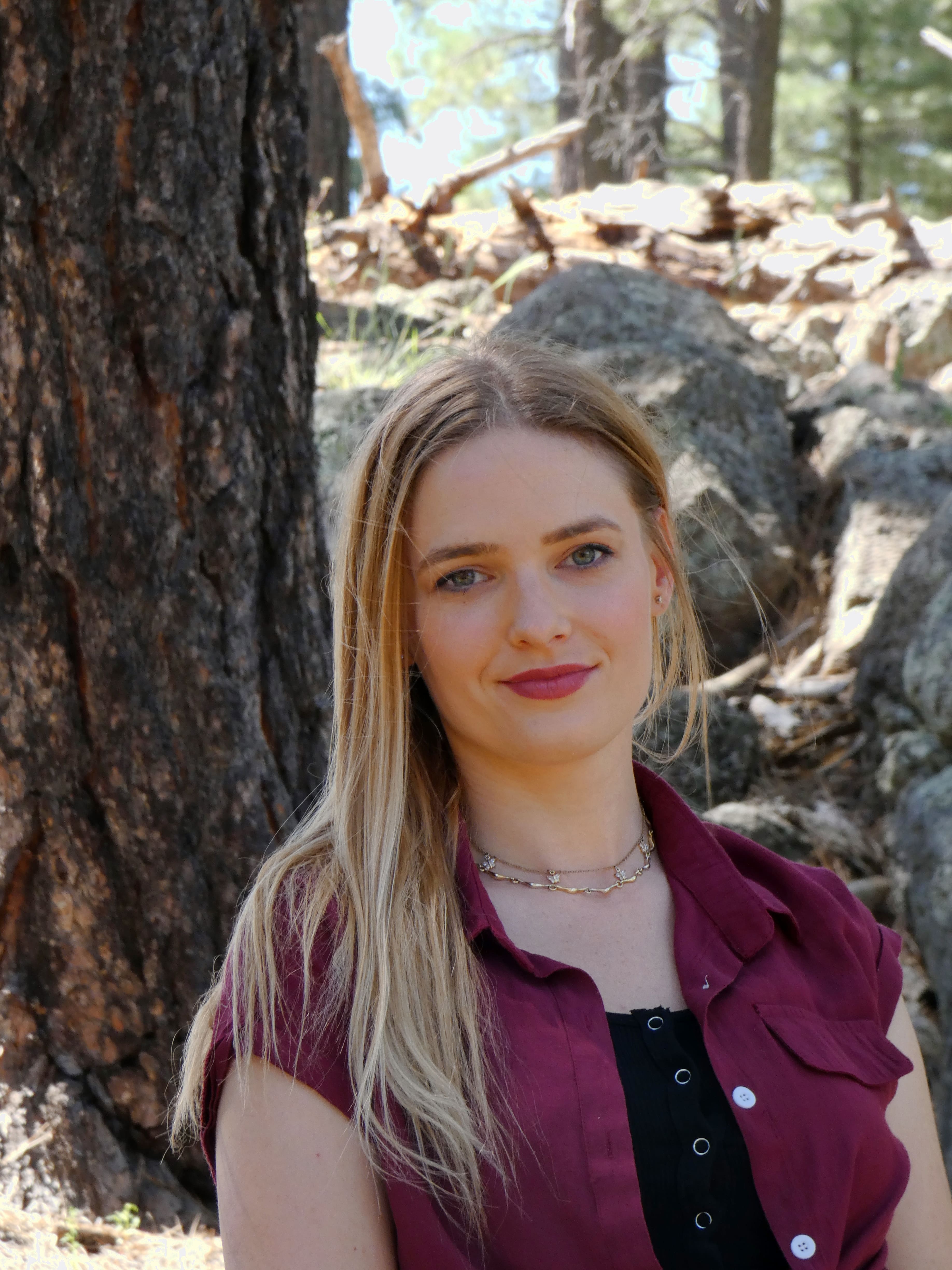
Tamara
SZENTIVANYI